Keeping cool: Record breaking on-chip thermometry
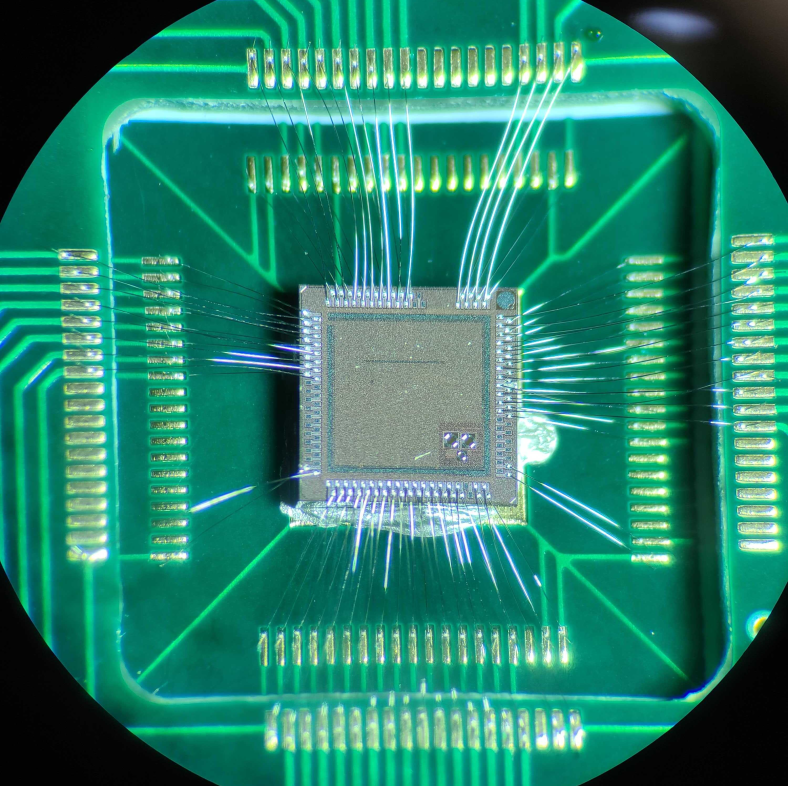
The microprocessors in your computer come embedded with temperature sensors, to ensure the CPU doesn’t overheat. Similar on-chip sensors need to be developed for the control electronics integrated into quantum computers, but these have the additional challenge of working at the ultra-low temperatures needed for quantum computing.
The majority of leading quantum processor platforms (spin, trapped ion, superconducting, and photonic qubits) demonstrate optimum behaviour at deep cryogenic temperatures (0.01 to 4 K). But the ‘classical’ digital and analogue electronics which are used for the control, addressing, and read-out of quantum processors dissipate power like any conventional circuit creating hotspots across chips.
Our teams are working on integrating control electronics with quantum processors. This means we also need to develop cryogenic ICs with power management solutions and on-chip thermometry methods capable of monitoring the temperature across chips operating at down to 10s of milliKelvin (mK).
To explore which types of on-chip thermometry would perform best — be the most sensitive and versatile enough to cover the full temperature range — our teams set up a competition between four different approaches. We adapted two existing ways in which temperature sensing is done on CMOS microprocessors, and tested two new ways that are only possible at cryogenic temperatures: exploiting the physical phenomena of superconductivity or Coulomb blockade which can be seen in CMOS chips at low temperatures.
In our recent paper published in Applied Physics Reviews, our team benchmarked these four methods of CMOS on-chip thermometry that operate at milliKelvin temperatures on our Bloomsbury chip. The winner of our “thermometer competition” was unexpected and discovered almost by accident!
Quantum dot thermometry
Quantum Engineer Mathieu de Kruijf: “When we were designing Bloomsbury, the quantum hardware team always had a plan to use field effect transistors (FETs) for quantum dot thermometry (QDT) to accurately map the on-chip temperature. Due to their small size, FETs can be used to trap individual electrons in a quantum dot formed in the silicon channel directly under the gate electrode. We can then use the fundamental physics behind the Coulomb blockade that occurs at cryogenic temperatures to extract the local temperature. This is a decades old process that is standard practice for physicists in research labs. It’s an example of primary thermometry, where the temperature measurement can be extracted from a physical principle without reference to another thermometer.”
The team found that QDT can be used to make measurements between 1.5 – 6 K. Below 1.5 K, they found that the reading starts to saturate due to fluctuating charges close to the quantum dot, and the thermometer becomes inaccurate and eventually plateaus at 1.12 K. However, they found that despite the advantage of being a primary thermometer (not requiring calibration), the sensitivity of QDT was comparatively poor with respect to the other methods.
Gate resistance thermometry
Staff IC Validation Engineer Grayson Noah: “In the IC team, we tried something new to measure temperature on Bloomsbury. We had seen several researchers using gate resistance thermometry (GRT) at room and cryogenic temperatures. At first glance, gate resistance doesn’t appear to be useful for deep-cryogenic thermometry because there is no sensitivity below around 30 Kelvin. However, even if the ambient temperature around your chip is 4 K or below, it’s very easy for your local on-chip temperature at a single device, if it’s a very power-heavy device, to be above 30 Kelvin, which is something we’ve seen if you put 100 microwatts or more into a single transistor. While this method is not useful for quantum devices, it is useful to map hotspots in the classical circuitry and characterise large temperature gradients across a chip.”
The team found that GRT can be used to make measurements between 30 – 300 K. Here the sensitivity of the measurement is better than what can be achieved with QDT, but since this technique doesn’t work below 30 K, its primary use is to characterise self-heating, being an extremely local measure of the temperature in a given transistor device.
Superconducting phase transition thermometry
Superconducting thin film thermometry (SPTT) was not necessarily a technique the team had planned to use when they were designing the Bloomsbury chip. SPTT makes use of the behaviour of superconductors to measure temperature.
Quantum Engineer Tom Swift: “Due to the materials and design of Bloomsbury, we were expecting that some of the structures on the chip would become superconducting, but what we found really exciting was that material in the gates of Bloomsbury’s transistors also became superconducting, meaning we could do extremely low temperature thermometry on the gate of a transistor! This measurement also gave us more insight into the superconducting material of the gate stack itself!”
The team found that SPTT can be used to make measurements between 0.6 – 1.2 K. A greater level of sensitivity can be achieved with this technique when compared to GRT, but it is a harder technique to integrate on-chip due to design considerations and the additional independent circuit functions needed to make measurements.
Diode thermometry
Diode thermometry (DT) is a classic thermometry technique that electronics engineers use on ICs across the world for power management and thermal analysis. This wasn’t a thermometry method the team had initially planned to explore on Bloomsbury but was discovered almost by accident.
Staff IC Validation Engineer Grayson Noah: “Electro Static Discharge diodes are structures right by the pad where you bond to the chip, they are designed to protect the interior circuits from signal pulses that wouldn’t be safe for the interior circuits. So it’s a protective structure that’s generally there to keep you from accidentally damaging your internal circuits. But at the same time, they’re just regular diodes.”
“So when I did my basic continuity measurements, I would bias these diodes to make sure I was connected to the pad that I thought I was. And I noticed, of course, that there’s a big difference between cryo and room temperature voltages on these diodes. So then we started analysing, cooling down and warming up while looping the measurements on the diodes, and they behave as expected near room temperature, but then we can actually get even higher sensitivities at cryogenic temperatures.”
“We can control the sensitivities by tuning the current that we’re driving through the diode. We need a small current so that we don’t saturate with self heating of the diode itself. So with this small current, we’re able to do the lowest temperature diode thermometry measurements that we’ve seen published, exhibiting good sensitivity down to about 200 or 150 milliKelvin, whereas every other publication we’ve seen hasn’t demonstrated utility below 1 Kelvin.”
Our team has shown that diode thermometry enables sensitive thermometry well below 1.5 K, the common lower limit for commercial cryogenic diode thermometers. They found that diode thermometry can have significant temperature sensitivity maintained down to 150 mK. We found that diode thermometry, particularly when biased with a low current, is the most sensitive method when compared to the other methods across the whole temperature range studied (20 mK to 300 K). We envision that the sensitivity of the diode thermometry technique could be improved further by operating the sensor in conjunction with fast readout techniques.
All the methods explored by the team have the ability to be integrated in scaled-up circuits, helping us build towards our goal of a scalable quantum silicon processor.
Developing a temperature map
Alberto Gómez Saiz, Principal IC Design Engineer : “Our next goal is to further develop a temperature map from a point heat source on a chip. We already have a good basis from the thermal modelling work we have already completed. This mapping would give us a way to predict the temperature at a given distance from a heat source. So for example, if you have in the same chip a controlling circuit and a quantum dot structure, you would know how far apart they need to be to ensure that the quantum properties don’t get degraded due to an elevated temperature.”
Understanding temperature is critical when designing cryogenic and quantum ICs, our teams all work together – from modelling, IC design and validation to quantum hardware – to design CMOS quantum chips. If you want to be part of this revolution in silicon computing take a look at our careers section. We are always looking to speak to talented people – even if we don’t have your dream role listed, please get in touch via jobs@quantummotion.tech.